Almost every organism, from the simplest virus to the complex human, possesses genetic material such as DNA or RNA. DNA itself is nothing but a sequential arrangement of the nitrogen base pairs. A specific segment of this DNA, called as the gene, serves as the functional unit of heredity. These genes determine various traits, from eye colour to disease susceptibility. What’s intriguing is that even a minor spontaneously occurring change in the sequence of these genes can have major repercussions. Initially, some changes might go unnoticed, but they accumulate over time, leading to genetic disorders or evolutionary adaptations.
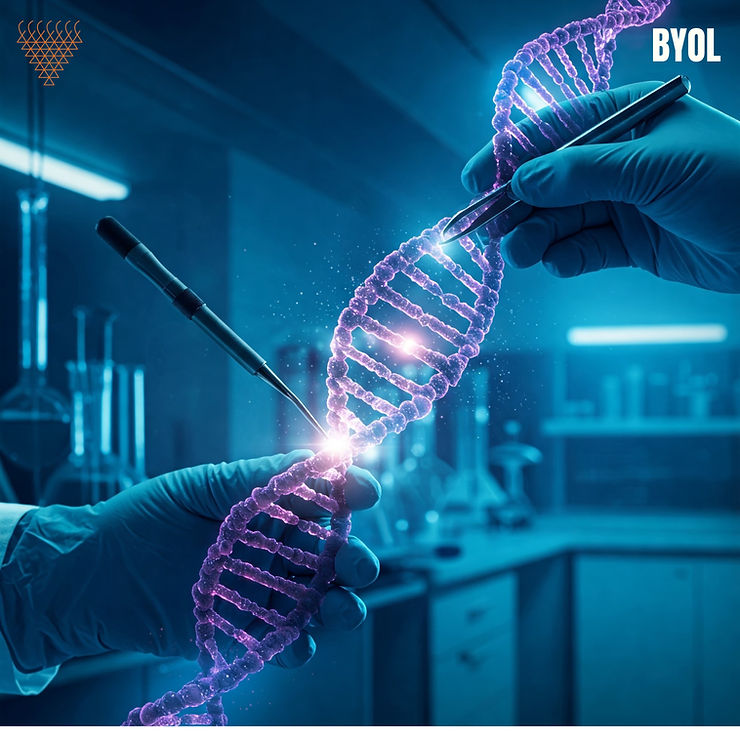
But what if we could take control of these changes? What if we could precisely edit these genes, remove mutations, or even add some, thereby unlocking the ability to combat diseases at their very source? CRISPR [Clustered regularly interspaced short palindromic repeats], a groundbreaking gene editing tool, has made this possible. It has transformed genetic research and stands at the forefront of one of the most exciting, yet controversial scientific advancements of our time.
What is CRISPR?
CRISPR stands for Clustered Regularly Inter Spaced Palindromic Repeats. These are short repeated sequences of DNA found in the prokaryotes. Since they read the same way forward and backwards like the English words civic or refer, they were termed as Palindromes.
These palindromic sequences of DNA repeat themselves after certain base pairs. These base pairs present between the palindromes are called as spacers. So, it goes like a palindrome followed by a spacer then a palindrome and so on. These spacers are bits of foreign DNA that traditionally do not belong to the bacterium. The bacteria knicks off certain fragments of viral DNA when it attacks, and incorporates them as spacers. Thus, spacers are a record of past infection, aiding in adaptive immunity. The CRISPR sequence is flanked by genes that get transcribed and are further translated into enzymes. These enzymes are called as CRISPR-associated or Cas enzymes. The most researched Cas enzyme is Cas9.
Once the bacteria incorporates the spacers, the central dogma of molecular biology is followed. The entire CRISPR array gets transcribed into an RNA called as the precursor crRNA [CRISPR RNA].A part of the DNA usually located near the Cas gene codes for a non-coding RNA called as trans-activating CRISPR RNA (transcrRNA). These RNA are complementary to the repeats in the pre-crRNA and can anneal to it. This facilitates the cleavage of pre-crRNA into individual crRNA by Ribonuclease III. So, after transcription of the DNA, we get a complex containing a crRNA, a tracrRNA and a Cas enzyme.
During reinfection, this complex identifies a viral DNA complementary to the crRNA. The Cas enzyme-crRNA complex recognizes a protospacer adjacent motif. This is a short sequence unique to the virus, which enables the Cas to recognize the target and cleave it. The virus is thus unable to replicate and the threat is neutralized.
History of CRISPR
The discovery of CRISPR and its incorporation as a gene editing tool was the culmination of the collaborative effort of the scientific community over decades.
In 1986, while sequencing the gene of an E.coli bacteria at Osaka University in Japan, Yoshizumi Ishino stumbled upon a sequence of 29 nucleotides arranged as direct repeats. These repeats were found in many other prokaryotic species. However, it was not until late 2001 when Francisco Mojica and Rudd Jansen coined the acronym CRISPR(clustered regularly interspaced palindromic repeats) for these sequences. These repeats were flanked by genes that directed the coding for specific enzymes, later termed CRISPR-associated or Cas enzymes. Mojica went on to discover similar sequences from the genomes of bacteriophages (the virus that attacks bacteria). Thus, he hypothesized that CRISPR is an adaptive immune system. However, the mechanism of these sequences remained a mystery.
Alexander Bolotin in 2005 discovered Cas9, which had nuclease property. While studying Streptococcus thermophilus, Bolotin identified that the spacers that had homology to the virus had a common sequence at the end. The sequence was termed the protospacer adjacent motif or PAM, which is required for target recognition.
In 2005,Rodolphe Barangou and Phillppe Horvath demonstrated that bacteria incorporated certain fragments of viral DNA as spacers into their genome after an attack. These sequences serve as a memory, enabling the bacteria to recognize and prevent future attacks by the same virus.
It was John van der Oost who identified that the bacteria made RNA copies of these spacers. These RNAs were termed as CRISPR RNAs (crRNAs). Marraffini and Erik Sontheimer deciphered that the target of the CRISPR system is DNA. Emmanuelle Charpentier finished the puzzle by discovering trans-activating CRISPR RNA (tracrRNA).
From an Adaptive Immune Response to a Programmable Gene Editing Tool
Once the scientists intricately understood the functioning of CRISPR systems, they demonstrated that it could be reprogrammed to target a site of our choice, by changing the sequence of the crRNA. In 2012, Jennifer Doudna at the University of California Berkeley with Emmanuelle Charpentier simplified the system by fusing the crRNA and the tracrRNA into a single guide RNA. Scientists could now design their own single guide RNA of up to 20 nucleotides as a target for editing. This sgRNA can be inserted into a cell along with Cas protein. The complex reads the DNA and cleaves both the strands at the desired location. The knicked DNA undergoes repair either by Homology-directed repair or Non-Homologous End joining (NHEJ). The programmable sgRNA allows us to precisely edit almost any gene at our desired location. For this remarkable gene editing toolkit, Jennifer Doudna and Emmanuelle Charpentier received the Nobel Prize in Chemistry in 2020.
Applications of CRISPR
Feng Zhang of the Broad Institute of MIT and Harvard demonstrated the action of CRISPR technology in human and mouse cells. They showed that the technology can be used to target multiple genetic loci. This unlocked the wide application potential of CRISPR Cas9.
The technology has applications in screening, diagnostics and therapeutics. Some of them are enumerated:
Blood Disorders: In December 2023, Casgevy was approved by the FDA. It is the first CRISPR-based therapy that modifies hematopoietic stem cells for sickle cell disease.
Cancer therapeutics: T cells can be reprogrammed to eliminate cancer. In 2022, it was successfully employed in a British girl with Acute lymphoblastic leukaemia.
HIV Cure: CRISPR with antiretroviral therapy has been instrumental in making the virus undetectable in humanized mice. However, further evaluation is warranted.
SARS CoV2: The technology is used for detection of SARS-CoV-2. The diagnostic tools are SHERLOCK and AIOD-CRISPR.
Agriculture: Since 2013, it has been employed to improve crop traits and disease resistance.
The application of the CRISPR system is also being explored in various other fields like blindness, familial hypercholesterolemia, type 1 diabetes, genetic anthropology, gene therapy and infectious diseases.
Ethical dilemma
In November 2018, Jiankui HI announced the editing of two human embryos to disable the gene CCR5 which plays a role in susceptibility to HIV. However, the twin girls Lulu and Nana, still carried functional copies of CCR5, making them vulnerable.His work was widely condemned as unethical, premature, and unsafe, as the technology is not ready for application in reproductive medicine.
While CRISPR is a very promising tool, it can be misused for germline genome modification.It can lead to ethical dilemmas for Designer Babies. The ability to choose traits for future generations raises questions about limits of genetic intervention and the balance between the role of humans and nature in shaping evolution.
There is an urgent need to ensure strict ethical guidelines and regulations for the moral advancement of CRISPR Cas9 technology.
References:
- Cong, L., Ran, F.A., Cox, D., Lin, S., Barretto, R., Habib, N., Hsu, P.D., Wu, X., Jiang, W., Marraffini, L.A., et al. (2013). Multiplex genome engineering using CRISPR/Cas systems. Science 339, 819–823.
- Mojica, F.J.M., D ez-Villase or, C.S., Garc a-Mart nez, J.S., and Soria, E. (2005). Intervening Sequences of Regularly Spaced Prokaryotic Repeats Derive from Foreign Genetic Elements. J Mol Evol 60, 174–182
- “CRISPR Timeline”. Broad Institute. 2015-09-25.
- Commissioner, Office of the (2024-08-09). “FDA Approves First Gene Therapies to Treat Patients with Sickle Cell Disease”. FDA.
- Uddin F, Rudin CM, Sen T (August 2020). “CRISPR Gene Therapy: Applications, Limitations, and Implications for the Future”. Frontiers in Oncology. 10: 1387. doi:10.3389/fonc.2020.01387. PMC 7427626. PMID 32850447.
- Begley S (28 November 2018). “Amid uproar, Chinese scientist defends creating gene-edited babies”. STAT.