Wearable biosensors have emerged as a pivotal technology for the convenient monitoring of health issues and serve as a foundation for personalised healthcare. The devices assess many physical, functional, chemical, and biological factors (biomarkers) that can be employed to draw educated conclusions about a patient’s overall health status. More than 10,000 devices are categorised as physiological telemetry wearables, which track heart signals, body temperature, sleep data, and other vital signs. In addition to basic wearables, clinicians are progressively utilising sophisticated body sensors to subjectively evaluate patients in outpatient clinics and, conversely, to orchestrate extensive clinical studies in hospitals. The predominant use of body sensors today is for the routine monitoring of health conditions by specialists, general practitioners, and endocrinologists. The implementation of real-time monitoring of the human physiome by miniaturised nanotechnology-based wearable biosensors presents numerous potential advantages.
Progress in Wearable Biosensor Technology
- A standard biosensor comprises two essential functional components: a ‘bioreceptor’ (such as an enzyme, antibody, or DNA) that selectively identifies the target analyte, and a ‘physico-chemical transducer’ (including electrochemical, optical, or mechanical types) that transforms this biorecognition event into a meaningful signal. These sensors were initially developed for in vitro assessments in regulated environments like laboratories or for single-use home diagnostics (e.g., blood glucose test strips).
- Wearable biosensors have garnered significant attention over the past couple decades, resulting in substantial advancements in sensor design, materials, and fabrication techniques. In this context, micro- and nanotechnology advancements are generating extensive opportunities for the development of highly sensitive, selective, stable, reproducible, miniaturised, and integrated biosensor systems. In contrast, the increasing integration of biosensors with artificial intelligence, edge computing, big data management, metropolitan area network technologies, and other advanced technologies have facilitated the development of low-cost, user-friendly, and real-time monitoring and diagnostic devices. The Internet of Things (IoT) revolution, cost-effective electronics, wireless telecommunication systems, nanomaterials, nanofabrication, and printing technologies propel the miniaturisation of biosensors and enable straightforward, internet-based wireless communication.
Epidermal wearable biosensors
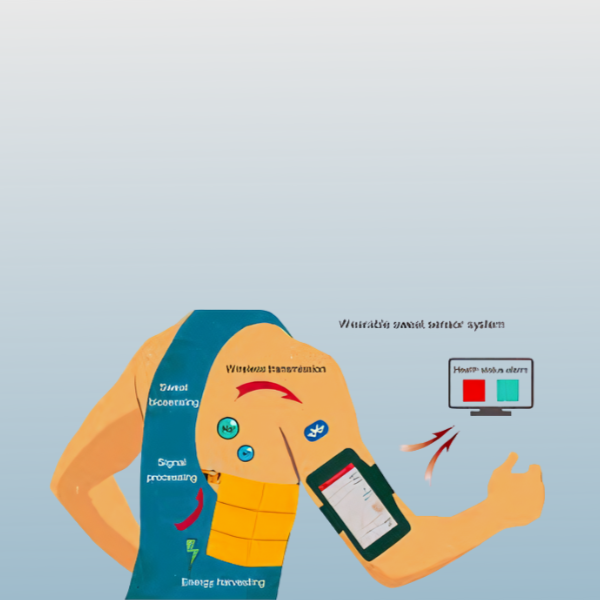
- Due to the epidermis encompassing a significant portion of the body, skin-worn conformal devices have recently attracted considerable interest among various types of wearable biosensors. Epidermal biosensors provide real-time biomarker detection in epidermal biofluids, such as sweat and interstitial fluid, with specific devices demonstrating continuous monitoring capabilities for various biomedical and fitness applications. These devices rely on the sampling of sweat or interstitial fluid from the skin surface and the subsequent transit of these biofluids across the biosensor surface. These dermal biosensors generally rely on multiple transduction modalities (e.g., optical, electrochemical, and mechanical) with biocatalytic and ion-recognition receptors.
- Recent advancements have predominantly focused on electrochemical and colorimetric transduction techniques. These devices have been developed through the direct application of sensors onto the skin (utilising E-skin or printed temporary tattoos), the incorporation of sensors into wristbands and patches, or the embedding of sensors within fabrics to ensure proximity to the skin while enduring the mechanical stress associated with bodily movements.
Wearable sweat biosensors based on exercise
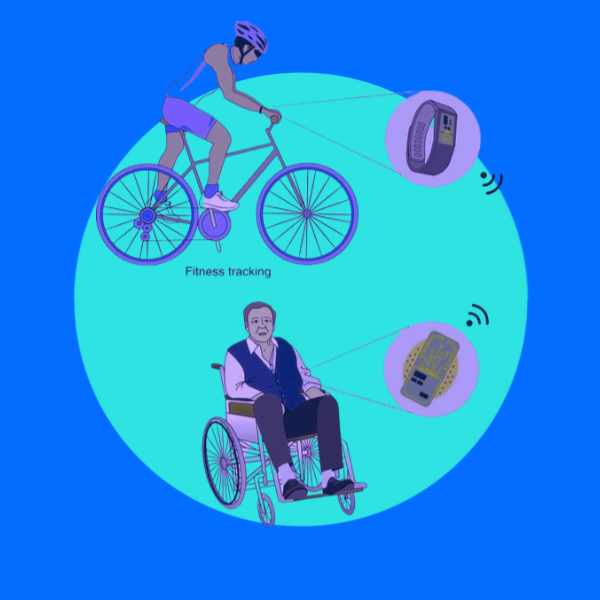
- Preliminary advancements in epidermal wearable biosensing devices were established as single-analyte detection, including a wide array of targeted analytes. These proof-of-concept examples were exhibited through innovative stress-resistant materials and sensor architectures to provide the requisite high level of skin conformability for effective sweat sampling during exercise, such as tattoo-type platforms. Flea-market tattoos, in conjunction with screen-printed flexible electrodes, present a highly promising foundation for skin-worn biosensing devices due to their ability to maintain direct and continuous contact with the skin surface. These body-compliant sensors integrate very desirable substrate skin flexibility and intimate skin contact with exceptional electrochemical performance.
- Tattoo epidermal biosensors have been shown to provide real-time, noninvasive measurement of key sweat electrolytes (pH, ammonium, or sodium), heavy metals (Zn), and metabolites (lactate or ethanol).
- Research has revealed real-time monitoring of sweat lactate concentrations utilising epidermal electrochemical biosensors, providing an immediate picture of lactate dynamics in sweat during physical exertion. Sweat lactate is a byproduct of the metabolism in local sweat glands, and intense physical activity enhances its production rate. Although sweat lactate does not directly correspond with concurrent blood concentrations, it indicates the intensity of physical exertion during prolonged exercise and can act as a metric for athletic efficiency without requiring an invasive blood sample.
- Recent studies have focused on broadening target biomarkers to include those related to hormonal and immunological responses. A wearable immunosensor for the detection of interleukin-6 and cortisol in sweat, with diagnostic consequences, is exemplified.
- Piezoelectric biosensing systems have emerged as innovative electronic-skin platforms for monitoring sweat metabolites. The produced piezoelectric signal is contingent upon body movement (exercise) and the concentration of the analyte in sweat. This produces a self-sustaining biosensor capable of distinguishing sweat analyte concentrations, hence obviating the need for an external power source or battery.
Epidermal biosensors utilising iontophoresis
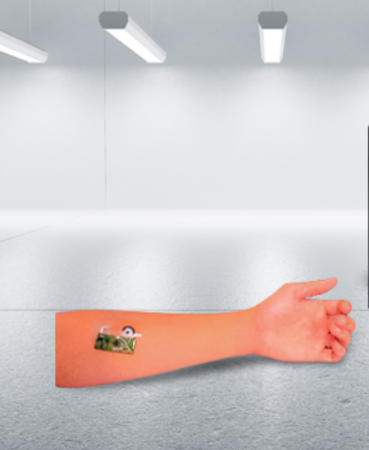
- Interstitial fluid and sweat epidermal biofluids can be acquired noninvasively by iontophoresis for substantial biomonitoring purposes. This procedure involves applying a mild current through the skin to facilitate ion migration between two electrodes placed on the skin.
- ISF can be acquired using reverse iontophoresis, which uses a low current to induce the movement of positively charged ions towards the negatively charged skin surface, alongside an electro-osmotic flow from anode to cathode. This flow also induces the migration of neutral substances, such as glucose, towards the cathode. Interstitial fluid glucose levels correctly represent blood glucose, as interstitial fluid components diffuse straight from capillary endothelial walls. Glucose levels in interstitial fluid can be easily quantified using glucose biosensors affixed to the skin.
- The inaugural presentation of a reverse iontophoresis-based sensing system was conducted by Cygnus, presenting a wearable wrist-mounted gadget called the GlucoWatch Biographer. This FDA-approved device facilitates noninvasive glucose monitoring for 12 hours, providing six measures each hour.
Ocular Wearable Biosensors
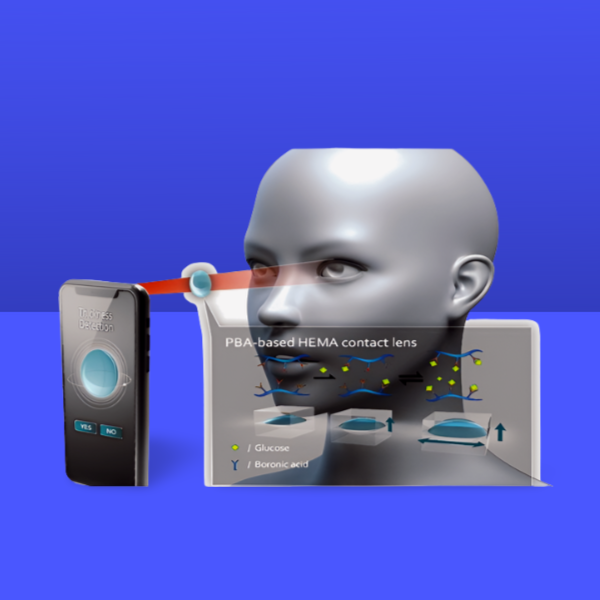
- Tears represent an additional bodily fluid that can be utilised to assess physiological status. Biomarker chemicals in tears diffuse from the blood stream establishing direct tear-blood concentration correlations, and enables the detection of ocular diseases.
- Tears are less complex than blood and are part of the eye’s antifouling mechanism. These characteristics make human tears a viable diagnostic biofluid for non-invasive healthcare monitoring applications.
Wearable biosensors based on tears
- Contact lens-based devices present an attractive option for tear collection issues, as they may be worn comfortably without causing eye irritation and maintain continuous direct contact with basal tears. These devices integrate all necessary biosensing, data processing, and power sources into the contact lens platform, which might provide challenging design specifications.
- Contact lens-based sensors were initially suggested for the optical measurement of tear glucose, relying on the interaction between glucose and concanavalin A or phenylboronic acid derivatives. The feasibility of quantitatively measuring glucose in human tear fluid under physiological conditions using holographic contact lenses was proposed concurrently, highlighting advantages such as the elimination of a battery, ease of reading, and continuous glucose signal monitoring.
- Additional optical sensors utilising contact lenses have used photonic crystal materials alongside responsive hydrogels or fluorescent dyes to identify glucose and other specific analytes in tears. The amalgamation of optical sensors with smartphone-based microscopes, incorporating algorithm-driven software, is anticipated to facilitate the interpretation of the biosensor response.
- Recently, ‘smart’ contact lenses for wireless ocular diagnostics have been advanced by merging glucose and ocular pressure sensors to provide wireless in vivo glucose monitoring in a rabbit’s eye alongside in vitro monitoring of ocular pressure using a bovine eyeball.
- In addition to contact lens platforms, NovioSense developed a miniature spring-like electrochemical sensor, consisting of multiple coiled wire electrodes encased in a protective polysaccharide-based hydrogel, designed for insertion into the inferior conjunctival fornix to maintain continuous access to tear fluid. The positioning of the sensor at the bottom of the eye, under the eyelid, guarantees uninterrupted glucose monitoring in tears, facilitated by wireless data transmission without intrusiveness.
Oral Cavity Wearable Biosensors
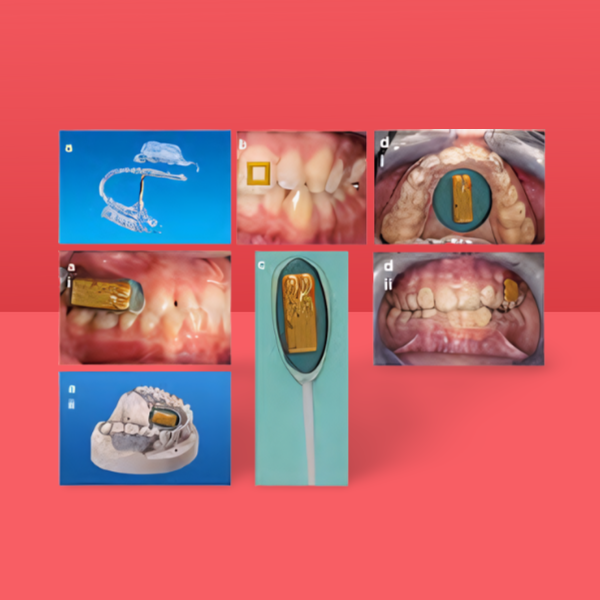
The interest in saliva as a diagnostic fluid has rapidly advanced in recent years. Numerous indicators in saliva originate directly from the bloodstream via transcellular or paracellular pathways, rendering saliva a ‘mirror of the human body’ that reflects physiological conditions and offers a noninvasive alternative to blood examination. Saliva, being readily accessible, has been employed in conjunction with in vitro diagnostic biosensors on strip or handheld device platforms.
Wearable biosensors utilising saliva
- The earliest known wearable oral sensor was introduced in the 1960s and employed a partial denture platform to detect mastication, plaque pH, and fluoride concentrations. This intraoral procedure, however, required the substitution of several teeth with sensors and was laden with hazards due to the potential leakage of the internal sensor fluid.
- Mannoor et al. expanded the oral biosensing domain by demonstrating graphene-based nanosensors printed on water-soluble silk and immediately transferred onto tooth enamel for passive, wireless bacterial detection. The oral-cavity sensors were integrated with a resonant coil for battery-free operation and could detect salivary bacteria at the single-cell level in vitro by employing a naturally occurring biorecognition element from an antimicrobial peptide, along with label-free impedance transduction. This attractive wearable biosensing concept was designed for the remote detection of bacterial biofilm formation on teeth and might be used for the broader detection of other salivary indicators.
- Studies have revealed the development of oral-cavity sensors by demonstrating a mouthguard-based uric acid biosensor that incorporates anatomically miniaturised electronic instrumentation, a potentiostat, a microcontroller, and a Bluetooth low energy (BLE) transceiver to monitor salivary uric acid concentration for potential clinical applications. This system facilitates noninvasive monitoring of salivary uric acid as a proxy for blood uric acid, a biomarker for various diseases (e.g., hyperuricemia, gout, and renal syndrome).
- Oral biosensing devices have been further miniaturised into a removable ‘cavitas sensor’ device to monitor salivary glucose on a mouthguard platform designed to conform to the wearer’s teeth.
Power challenges of wearable biosensors
Power consumption in most wearable biosensors originates from three primary sources:
- Energy required for biomarker-detector sensors, which increases with multiplexing.
- Data processing which balances data collection rate and energy expenditure.
- Wireless communication encompasses interactions with other integrated sensors and data transmission.
Successfully meeting these power requirements entails multiple approaches, like:
- Secure wearable high-energy batteries.
- Alternative power harvesting and storage devices such as biofuel cells, solar cells, thermoelectric systems, piezoelectric devices, triboelectric generators, supercapacitors, or a combination of multiple sources.
- Wireless power transmission.
- Energy-autonomous sensing devices.
- Biosensors powered by biofuel cells utilising the analyte as fuel.
Communication challenges of wearable biosensors
- To realise their full potential, wearable biosensors must facilitate wireless communication among individual users, multiplexed biosensors, and computing devices. They must also possess the capability to transport data across extensive distances while achieving these goals in an energy-efficient manner.
- Wireless communication strategies include:
- Bluetooth, particularly Bluetooth Low Energy (BLE), which offers reduced power consumption but is limited to a 100-meter reception range.
- Near field communication (NFC) utilising radio frequency identification (RFID), which is battery-free but requires proximity for signal reception.
Data protection in wearable biosensors
- Wearable biosensors present novel security threats. A primary benefit of wearable biosensors for medical applications is their ability to monitor patients’ health status in real time, remotely, and continuously. Nonetheless, these potent characteristics could jeopardise consumers if hardware and software infrastructures are not designed with privacy and security in mind. Access to individuals’ biomedical information must be rigorously regulated by authorised personnel to avert privacy violations.
- The threat of an external assault can be mitigated with reliable authentication and encryption techniques. Data protection vulnerabilities are particularly severe during an insider attack by persons possessing legitimate access to raw data. The assailant can input erroneous health information that may affect a patient’s diagnosis and treatment. The prompt identification of such intrusions will mitigate security threats and uphold the confidentiality, reliability, and integrity of healthcare information generated by wearable biosensors.
- The substantial volume of data produced by continuous real-time measurements from these biosensors requires the implementation of sophisticated data processing protocols. Common techniques applied to data from wearables include data cleaning and filtering processes, such as noise filtering, which can impair wireless data transfer rates and energy efficiency.
- Furthermore, advanced data mining algorithms can predict potential hazardous conditions and establish connections between sensor signals and clinical diagnostics within a substantial population of wearers.
Transference to the commercial sector
The global wearable sensors market was valued at around $150 million in 2016 and is projected to expand to $2.86 billion by 2025, according to a recent market analysis. A significant portion of this potential market share will consist of wearable biosensors, primarily for noninvasive glucose monitoring. The slower-than-anticipated pace of noninvasive biosensing platform commercialisation has diminished market expectations; nevertheless, the rapid increase in research publications and proof-of-concept demonstrations has sustained optimism regarding the market potential of wearable biosensors.
Outlook
Wearable biosensors will progressively be engineered for greater integration, shifting from the wrist to clothing and fashion accessories that effortlessly blend into a wearer’s regular activities. Some of these devices will use disposable components to address fouling. Future wearable biosensors will noninvasively monitor a wide range of biomarkers, including proteins and nucleic acids, ultimately facilitating comprehensive medical diagnosis and performance assessment. The medical community’s endorsement of noninvasive biosensors will require extensive and effective validation through human studies, together with enhanced understanding of the therapeutic use of sensor data. Given the competitive analysis and significant commercial prospects of wearable biosensors, we anticipate exciting advances in the near future. The market for wearable sensors is positioned for sustained rapid growth, further altering and enhancing lives.
References
- Bandodkar, A. J., Jeerapan, I. & Wang, J. Wearable chemical sensors: present challenges and future prospects. ACS Sens. 1, 464–482 (2016).
- Heikenfeld, J. et al. Wearable sensors: modalities, challenges, and prospects. Lab Chip 18, 217–248 (2018).
- Matzeu, G., Florea, L. & Diamond, D. Advances in wearable chemical sensor design for monitoring biological fluids. Sens. Actuators B Chem. 211, 403–418 (2015).
- Liu, Y., Pharr, M. & Salvatore, G. A. Lab-on-skin: a review of flexible and stretchable electronics for wearable health monitoring. ACS Nano 11, 9614–9635 (2017).
- Amjadi, M., Kyung, K. U., Park, I. & Sitti, M. Stretchable, skin-mountable, and wearable strain sensors and their potential applications: a review. Adv. Funct. Mater. 26, 1678–1698 (2016).
- Bariya, M., Nyein, H. Y. Y. & Javey, A. Wearable sweat sensors. Nat. Electron. 1, 160–171 (2018).
- Kim, C.S. et al. Self-powered wearable electrocardiography using a wearable thermoelectric power generator. ACS Energy Lett. 3, 501–507 (2018).
- Bandodkar, A. J. et al. Soft, stretchable, high power density electronic skin-based biofuel cells for scavenging energy from human sweat. Energy Environ. Sci. 10, 1581–1589 (2017).
- Lee, Y. et al. Wireless, intraoral hybrid electronics for real-time quantification of sodium intake toward hypertension management. Proc. Natl Acad. Sci. USA 115, 5377–5382 (2018).